Impacts of climate change on energy systems in global and regional scenarios
Although our knowledge of climate change impacts on energy systems has increased substantially over the past few decades, there remains a lack of comprehensive overview of impacts across spatial scales. Here, we analyse results of 220 studies projecting climate impacts on energy systems globally and at the regional scale. Globally, a potential increase in cooling demand and decrease in heating demand can be anticipated, in contrast to slight decreases in hydropower and thermal energy capacity. Impacts at the regional scale are more mixed and relatively uncertain across regions, but strongest impacts are reported for South Asia and Latin America. Our assessment shows that climate impacts on energy systems at regional and global scales are uncertain due partly to the wide range of methods and non-harmonized datasets used. For a comprehensive assessment of climate impacts on energy, we propose a consistent multi-model assessment framework to support regional-to-global-scale energy planning.
This is a preview of subscription content, access via your institution
Access options
Access Nature and 54 other Nature Portfolio journals
Get Nature+, our best-value online-access subscription
cancel any time
Subscribe to this journal
Receive 12 digital issues and online access to articles
133,45 € per year
only 11,12 € per issue
Buy this article
- Purchase on SpringerLink
- Instant access to full article PDF
Prices may be subject to local taxes which are calculated during checkout
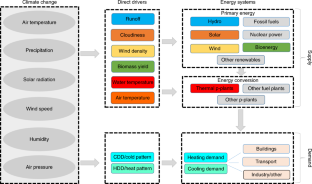
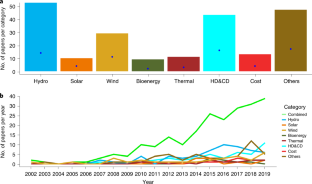
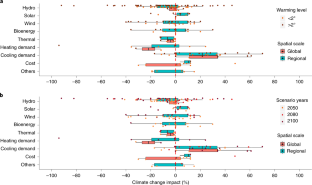
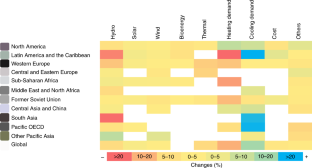
Similar content being viewed by others
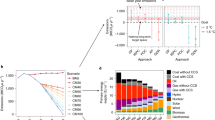
A framework for national scenarios with varying emission reductions
Article 27 May 2021
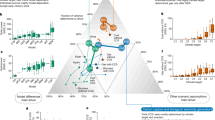
Spread in climate policy scenarios unravelled
Article Open access 13 December 2023
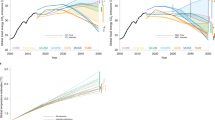
A multi-model analysis of long-term emissions and warming implications of current mitigation efforts
Article 22 November 2021
Data availability
All data that support the findings of this study presented in the figures are provided in the Source Data section associated with this manuscript. Source data are provided with this paper.
References
- Bruckner, T. et al. in Climate Change 2014: Mitigation of Climate Change (eds Edenhofer, O. et al.) (Cambridge Univ. Press, 2014).
- Schaeffer, R. et al. Energy sector vulnerability to climate change: a review. Energy38, 1–12 (2012). Google Scholar
- Ebinger, J. & Vergara, W. Climate Impacts on Energy Systems: Key Issues for Energy Sector Adaptation (The World Bank, 2011).
- Crook, J. A., Jones, L. A., Forster, P. M. & Crook, R. Climate change impacts on future photovoltaic and concentrated solar power energy output. Energ. Environ, Sci.4, 3101–3109 (2011). Google Scholar
- Owusu, P. A. & Asumadu-Sarkodie, S. A review of renewable energy sources, sustainability issues and climate change mitigation. Cogent. Eng.3, 1167990 (2016). Google Scholar
- Bartos, M. D. & Chester, M. V. Impacts of climate change on electric power supply in the Western United States. Nat. Clim. Change5, 748–752 (2015). Google Scholar
- Karnauskas, K. B., Lundquist, J. K. & Zhang, L. Southward shift of the global wind energy resource under high carbon dioxide emissions. Nar. Geosci.11, 38–43 (2018). Google Scholar
- Craig, M. T. et al. A review of the potential impacts of climate change on bulk power system planning and operations in the United States. Renew. Sust. Energ. Rev.98, 255–267 (2018). Google Scholar
- Van Vliet, M. T. et al. Vulnerability of US and European electricity supply to climate change. Nat. Clim. Change2, 676 (2012). Google Scholar
- Liu, L., Hejazi, M., Li, H., Forman, B. & Zhang, X. Vulnerability of US thermoelectric power generation to climate change when incorporating state-level environmental regulations. Nat. Ener.2, 17109 (2017). Google Scholar
- Ciscar, J.-C. & Dowling, P. Integrated assessment of climate impacts and adaptation in the energy sector. Energy Econ.46, 531–538 (2014). Google Scholar
- Ravestein, P., van der Schrier, G., Haarsma, R., Scheele, R. & van den Broek, M. Vulnerability of European intermittent renewable energy supply to climate change and climate variability. Renew. Sust. Ener. Rev.97, 497–508 (2018). Google Scholar
- Perera, A., Nik, V. M., Chen, D., Scartezzini, J. L. & Hong, T. Quantifying the impacts of climate change and extreme climate events on energy systems. Nat. Energy5, 150–159 (2020). Google Scholar
- IPCC Climate Change 2014: Synthesis Report (eds Core Writing Team, Pachauri, R. K. & Meyer L. A.) (IPCC, 2014).
- Isaac, M. & van Vuuren, D. P. Modeling global residential sector energy demand for heating and air conditioning in the context of climate change. Energy Policy37, 507–521 (2009). Google Scholar
- IPCC Climate Change 2014: Impacts, Adaptation, and Vulnerability (eds Field, C. B. et al.) (Cambridge Univ. Press, 2014).
- Cinner, J. E. et al. Building adaptive capacity to climate change in tropical coastal communities. Nat. Clim. Change8, 177–123 (2018). Google Scholar
- Lumbroso, D., Woolhouse, G. & Jones, L. A review of the consideration of climate change in the planning of hydropower schemes in sub-Saharan Africa. Clim. Change133, 621–633 (2015). Google Scholar
- Kabir, E., Kumar, P., Kumar, S., Adelodun, A. A. & Kim, K.-H. Solar energy: potential and future prospects. Renew. Sust. Energ. Rev.82, 894–900 (2018). Google Scholar
- Pryor, S. & Barthelmie, R. Climate change impacts on wind energy: a review. Renew. Sust. Energ. Rev.14, 430–437 (2010). Google Scholar
- Berndes, G., Hoogwijk, M. & Van den Broek, R. The contribution of biomass in the future global energy supply: a review of 17 studies. Biomass Bioenerg.25, 1–28 (2003). Google Scholar
- Li, D. H., Yang, L. & Lam, J. C. Impact of climate change on energy use in the built environment in different climate zones–a review. Energy42, 103–112 (2012). Google Scholar
- Auffhammer, M. & Mansur, E. T. Measuring climatic impacts on energy consumption: a review of the empirical literature. Energy Econ.46, 522–530 (2014). Google Scholar
- Mideksa, T. K. & Kallbekken, S. The impact of climate change on the electricity market: a review. Energy Policy38, 3579–3585 (2010). Google Scholar
- Chandramowli, S. N. & Felder, F. A. Impact of climate change on electricity systems and markets–a review of models and forecasts. Sust. Energ. Technol. Assess.5, 62–74 (2014). Google Scholar
- Mikellidou, C. V., Shakou, L. M., Boustras, G. & Dimopoulos, C. Energy critical infrastructures at risk from climate change: a state of the art review. Safety Science110, https://doi.org/10.1016/j.ssci.2017.12.022 (2017).
- Stanton, M. C. B., Dessai, S. & Paavola, J. A systematic review of the impacts of climate variability and change on electricity systems in Europe. Energy109, 1148–1159 (2016). Google Scholar
- Cronin, J., Anandarajah, G. & Dessens, O. Climate change impacts on the energy system: a review of trends and gaps. Clim. Change151, 79–93 (2018). Google Scholar
- Barnett, T. P., Adam, J. C. & Lettenmaier, D. P. Potential impacts of a warming climate on water availability in snow-dominated regions. Nature438, 303 (2005). Google Scholar
- Chilkoti, V., Bolisetti, T. & Balachandar, R. Climate change impact assessment on hydropower generation using multi-model climate ensemble. Renew. Energ.109, 510–517 (2017). Google Scholar
- Fan, J.-L. Impacts of climate change on hydropower generation in China. Comput. Simulat.167, 4–18 (2018). MathSciNetGoogle Scholar
- Teotónio, C., Fortes, P., Roebeling, P., Rodriguez, M. & Robaina-Alves, M. Assessing the impacts of climate change on hydropower generation and the power sector in Portugal: a partial equilibrium approach. Renew. Sust. Energ. Rev.74, 788–799 (2017). Google Scholar
- Hamududu, B. & Killingtveit, A. Assessing climate change impacts on global hydropower. Energies5, 305–322 (2012). Google Scholar
- Van Vliet, M. T., Wiberg, D., Leduc, S. & Riahi, K. Power-generation system vulnerability and adaptation to changes in climate and water resources. Nat. Clim. Change6, 375–380 (2016). Google Scholar
- Van Vliet, M. et al. Multi-model assessment of global hydropower and cooling water discharge potential under climate change. Global Environ. Change40, 156–170 (2016). Google Scholar
- Turner, S. W., Ng, J. Y. & Galelli, S. Examining global electricity supply vulnerability to climate change using a high-fidelity hydropower dam model. Sci. Total Environ.590, 663–675 (2017). Google Scholar
- Zhou, Y. et al. A comprehensive view of global potential for hydro-generated electricity. Energ. Environ. Sci.8, 2622–2633 (2015). Google Scholar
- Raje, D. & Mujumdar, P. Reservoir performance under uncertainty in hydrologic impacts of climate change. Adv. Water Resour.33, 312–326 (2010). Google Scholar
- Gaudard, L., Gilli, M. & Romerio, F. Climate change impacts on hydropower management. Water Resource. Manag.27, 5143–5156 (2013). Google Scholar
- Mohor, G. S., Rodriguez, D. A., Tomasella, J. & Júnior, J. L. S. Exploratory analyses for the assessment of climate change impacts on the energy production in an Amazon run-of-river hydropower plant. J. Hydrol. Reg. Studies4, 41–59 (2015). Google Scholar
- Fant, C., Schlosser, C. A. & Strzepek, K. The impact of climate change on wind and solar resources in southern Africa. Appl. Energy161, 556–564 (2016). Google Scholar
- Wachsmuth, J. et al. How will renewable power generation be affected by climate change? The case of a Metropolitan Region in Northwest Germany. Energy58, 192–201 (2013). Google Scholar
- Jerez, S. et al. The impact of climate change on photovoltaic power generation in Europe. Nat. Commun.6, 10014 (2015). Google Scholar
- Bartók, B. et al. Projected changes in surface solar radiation in CMIP5 global climate models and in EURO-CORDEX regional climate models for Europe. Climate Dynam.49, 2665–2683 (2017). Google Scholar
- Davy, R., Gnatiuk, N., Pettersson, L. & Bobylev, L. Climate change impacts on wind energy potential in the European domain with a focus on the Black Sea. Renew. Sust. Energ. Rev.81, 1652–1659 (2017). Google Scholar
- Carvalho, D., Rocha, A., Gómez-Gesteira, M. & Santos, C. S. Potential impacts of climate change on European wind energy resource under the CMIP5 future climate projections. Renew. Energ.101, 29–40 (2017). Google Scholar
- Hueging, H., Haas, R., Born, K., Jacob, D. & Pinto, J. G. Regional changes in wind energy potential over Europe using regional climate model ensemble projections. J. Appl. Meteorol. Climatol.52, 903–917 (2013). Google Scholar
- Tobin, I. et al. Vulnerabilities and resilience of European power generation to 1.5 °C, 2 °C and 3 °C warming. Environ. Res. Lett.13, 044024 (2018). Google Scholar
- Vautard, R. et al. Regional climate model simulations indicate limited climatic impacts by operational and planned European wind farms. Nat. Commun.5, 3196 (2014). Google Scholar
- Jerez, S. et al. Future changes, or lack thereof, in the temporal variability of the combined wind-plus-solar power production in Europe. Renew. Energy139, 251–260 (2019). Google Scholar
- De Lucena, A. F. P., Szklo, A. S., Schaeffer, R. & Dutra, R. M. The vulnerability of wind power to climate change in Brazil. Renew. Energy35, 904–912 (2010). Google Scholar
- Pereira, E. B., Martins, F. R., Pes, M. P., da Cruz Segundo, E. I. & Lyra, Ad. A. The impacts of global climate changes on the wind power density in Brazil. Renew. Energy49, 107–110 (2013). Google Scholar
- Breslow, P. B. & Sailor, D. J. Vulnerability of wind power resources to climate change in the continental United States. Renew. Energy27, 585–598 (2002). Google Scholar
- Sailor, D. J., Smith, M. & Hart, M. Climate change implications for wind power resources in the Northwest United States. Renew. Energy33, 2393–2406 (2008). Google Scholar
- De Jong, P. et al. Estimating the impact of climate change on wind and solar energy in Brazil using a South American regional climate model. Renew. Energy141, 390–401 (2019). Google Scholar
- Tuck, G., Glendining, M. J., Smith, P., House, J. I. & Wattenbach, M. The potential distribution of bioenergy crops in Europe under present and future climate. Biomass Bioenerg.30, 183–197 (2006). Google Scholar
- Bellarby, J., Wattenbach, M., Tuck, G., Glendining, M. J. & Smith, P. The potential distribution of bioenergy crops in the UK under present and future climate. Biomass Bioenerg.34, 1935–1945 (2010). Google Scholar
- Harvey, M. & Pilgrim, S. The new competition for land: food, energy, and climate change. Food Policy36, S40–S51 (2011). Google Scholar
- Kyle, P., Müller, C., Calvin, K. & Thomson, A. Meeting the radiative forcing targets of the representative concentration pathways in a world with agricultural climate impacts. Earth’s Future2, 83–98 (2014). Google Scholar
- Miara, A., Vörösmarty, C. J., Stewart, R. J., Wollheim, W. M. & Rosenzweig, B. Riverine ecosystem services and the thermoelectric sector: strategic issues facing the Northeastern United States. Environ. Res. Lett.8, 025017 (2013). Google Scholar
- Miara, A. et al. Climate and water resource change impacts and adaptation potential for US power supply. Nat. Clim. Change7, 793–798 (2017). Google Scholar
- Miara, A. et al. Climate-water adaptation for future US electricity infrastructure. Environ. Sci. Technol.53, 14029–14040 (2019). Google Scholar
- Byers, E., Hall, J., Amezaga, J., O’Donnell, G. & Leathard, A. Water and climate risks to power generation with carbon capture and storage. Environ. Res. Lett.11, 024011 (2016). Google Scholar
- Angeles, M. E., González, J. E. & Ramírez, N. Impacts of climate change on building energy demands in the intra-Americas region. Theoret. Appl. Climatol133, 59–72 (2018). Google Scholar
- Fan, J.-L., Hu, J.-W. & Zhang, X. Impacts of climate change on electricity demand in China: an empirical estimation based on panel data. Energy170, 880–888 (2019). Google Scholar
- Taseska, V., Markovska, N. & Callaway, J. M. Evaluation of climate change impacts on energy demand. Energy48, 88–95 (2012). Google Scholar
- Allen, M. R., Fernandez, S. J., Fu, J. S. & Olama, M. M. Impacts of climate change on sub-regional electricity demand and distribution in the southern United States. Nat. Energy1, 16103 (2016). Google Scholar
- Zhou, Y. et al. Modeling the effect of climate change on US state-level buildings energy demands in an integrated assessment framework. Appl. Energy113, 1077–1088 (2014). Google Scholar
- Hadley, S. W., Erickson Iii, D. J., Hernandez, J. L., Broniak, C. T. & Blasing, T. J. Responses of energy use to climate change: a climate modeling study. Geophys. Res. Lett.33, https://doi.org/10.1029/2006GL026652 (2006).
- Eom, J., Clarke, L., Kim, S. H., Kyle, P. & Patel, P. China’s building energy demand: long-term implications from a detailed assessment. Energy46, 405–419 (2012). Google Scholar
- McFarland, J. et al. Impacts of rising air temperatures and emissions mitigation on electricity demand and supply in the United States: a multi-model comparison. Clim. Change131, 111–125 (2015). Google Scholar
- Clarke, L. et al. Effects of long-term climate change on global building energy expenditures. Energy Econ.72, 667–677 (2018). Google Scholar
- Labriet, M. et al. Worldwide impacts of climate change on energy for heating and cooling. Mit. Adapt. Strat. Global Change20, 1111–1136 (2015). Google Scholar
- Van Ruijven, B. J., De Cian, E. & Wing, I. S. Amplification of future energy demand growth due to climate change. Nat. Commun.10, 2762 (2019). Google Scholar
- De Cian, E. & Wing, I. S. Global energy consumption in a warming climate. Environ. Res. Econ.72, 365–410 (2019). Google Scholar
- Auffhammer, M., Baylis, P. & Hausman, C. H. Climate change is projected to have severe impacts on the frequency and intensity of peak electricity demand across the United States. Proc. Natl Acad. Sci. USA114, 1886–1891 (2017). Google Scholar
- De Cian, E., Lanzi, E. & Roson, R. Seasonal temperature variations and energy demand. Clim. Change116, 805–825 (2013). Google Scholar
- Invidiata, A. & Ghisi, E. Impact of climate change on heating and cooling energy demand in houses in Brazil. Energ. Buildings130, 20–32 (2016). Google Scholar
- Wang, H. & Chen, Q. Impact of climate change heating and cooling energy use in buildings in the United States. Energ. Buildings82, 428–436 (2014). Google Scholar
- Hamlet, A. F., Lee, S.-Y., Mickelson, K. E. & Elsner, M. M. Effects of projected climate change on energy supply and demand in the Pacific Northwest and Washington State. Clim. Change102, 103–128 (2010). Google Scholar
- Davis, L. W. & Gertler, P. J. Contribution of air conditioning adoption to future energy use under global warming. Proc. Natl Acad. Sci. USA112, 5962–5967 (2015). Google Scholar
- Park, C. et al. Avoided economic impacts of energy demand changes by 1.5 and 2 °C climate stabilization. Environ. Res. Lett.13, 045010 (2018). Google Scholar
- Waite, M. et al. Global trends in urban electricity demands for cooling and heating. Energy127, 786–802 (2017). Google Scholar
- Morakinyo, T. E. et al. Estimates of the impact of extreme heat events on cooling energy demand in Hong Kong. Renew. Energy142, 73–84 (2019). Google Scholar
- Moazami, A., Nik, V. M., Carlucci, S. & Geving, S. Impacts of future weather data typology on building energy performance–Investigating long-term patterns of climate change and extreme weather conditions. Appl. Energy238, 696–720 (2019). Google Scholar
- Dirks, J. A. et al. Impacts of climate change on energy consumption and peak demand in buildings: a detailed regional approach. Energy79, 20–32 (2015). Google Scholar
- D’Oca, S., Hong, T. & Langevin, J. The human dimensions of energy use in buildings: a review. Renew. Sust. Energy Rev.81, 731–742 (2018). Google Scholar
- Poortinga, W., Steg, L. & Vlek, C. Values, environmental concern, and environmental behavior: a study into household energy use. Environ. Behav.36, 70–93 (2004). Google Scholar
- De Cian, E., Pavanello, F., Randazzo, T., Mistry, M. N. & Davide, M. Households’ adaptation in a warming climate. Air conditioning and thermal insulation choices. Environ. Sci. Policy100, 136–157 (2019). Google Scholar
- Castleton, H. F., Stovin, V., Beck, S. B. & Davison, J. B. Green roofs; building energy savings and the potential for retrofit. Energy Buildings42, 1582–1591 (2010). Google Scholar
- Jones, P., Lannon, S. & Patterson, J. Retrofitting existing housing: how far, how much? Building Res. Info.41, 532–550 (2013). Google Scholar
- Da Silva Soito, J. L. & Freitas, M. A. V. J. R. Amazon and the expansion of hydropower in Brazil: vulnerability, impacts and possibilities for adaptation to global climate change. Renew. Sust. Energy Rev.15, 3165–3177 (2011). Google Scholar
- Cohen, S. M., Macknick, J., Averyt, K. & Meldrum, J. Modeling Climate-Water Impacts on Electricity Sector Capacity Expansion (National Renewable Energy Laboratory, 2014).
- Mima, S. & Criqui, P. The costs of climate change for the European energy system, an assessment with the POLES model. Environ. Model. Ass.20, 303–319 (2015). Google Scholar
- Ackerman, F. & Stanton, E. A. The Cost of Climate Change: What We’ll Pay if Global Warming Continues Unchecked (Natural Resources Defence Council, 2008).
- Turner, S. W., Hejazi, M., Kim, S. H., Clarke, L. & Edmonds, J. Climate impacts on hydropower and consequences for global electricity supply investment needs. Energy141, 2081–2090 (2017). Google Scholar
- Van der Linden, P. & Mitchell, J. (eds) ENSEMBLES: Climate Change and its Impacts—Summary of Research and Results from the ENSEMBLES Project (European Environment Agency, 2009).
- Rübbelke, D. & Vögele, S. Impacts of climate change on European critical infrastructures: the case of the power sector. Environ. Sci. Policy14, 53–63 (2011). Google Scholar
- Pryor, S. & Barthelmie, R. Assessing the vulnerability of wind energy to climate change and extreme events. Clim. Change121, 79–91 (2013). Google Scholar
- Miller, N. L., Hayhoe, K., Jin, J. & Auffhammer, M. Climate, extreme heat, and electricity demand in California. J. Appl. Meteorol. Climatol.47, 1834–1844 (2008). Google Scholar
- Forzieri, G. et al. Escalating impacts of climate extremes on critical infrastructures in Europe. Global Environ. Change48, 97–107 (2018). Google Scholar
- Bartos, M. et al. Impacts of rising air temperatures on electric transmission ampacity and peak electricity load in the United States. Environ. Res. Lett.11, 114008 (2016). Google Scholar
- Panteli, M. & Mancarella, P. Influence of extreme weather and climate change on the resilience of power systems: Impacts and possible mitigation strategies. Electric Power Syst. Res.127, 259–270 (2015). Google Scholar
- Dowling, P. The impact of climate change on the European energy system. Energy Policy60, 406–417 (2013). Google Scholar
- Haddeland, I. et al. Multimodel estimate of the global terrestrial water balance: setup and first results. J. Hydrometeorol.12, 869–884 (2011). Google Scholar
- Schewe, J. et al. Multimodel assessment of water scarcity under climate change. Proc. Natl Acad. Sci. USA111, 3245–3250 (2014). Google Scholar
- Rosenzweig, C. et al. The agricultural model intercomparison and improvement project (AgMIP): protocols and pilot studies. Agri. Forest Meteorol.170, 166–182 (2013). Google Scholar
- van Vuuren, D. P. & Carter, T. R. Climate and socio-economic scenarios for climate change research and assessment: reconciling the new with the old. Clim. Change122, 415–429 (2014). Google Scholar
- Van Vuuren, D. P. et al. A new scenario framework for climate change research: scenario matrix architecture. Clim. Change122, 373–386 (2014). Google Scholar
- O’Neill, B. C. et al. A new scenario framework for climate change research: the concept of shared socioeconomic pathways. Clim. Change122, 387–400 (2014). Google Scholar
- Wiedenhofer, D., Lenzen, M. & Steinberger, J. K. Energy requirements of consumption: urban form, climatic and socio-economic factors, rebounds and their policy implications. Energy Policy63, 696–707 (2013). Google Scholar
- Frieler, K. et al. Assessing the impacts of 1.5 C global warming–simulation protocol of the Inter-Sectoral Impact Model Intercomparison Project (ISIMIP2b). Geosci. Model Dev.10, 4321–4345 (2017). Google Scholar
Acknowledgements
We wish to thank the JPI Climate initiative and participating grant institutes for funding the ISIpedia project. We also thank J. Burrough for professional advice on the English of a near-final draft. E.d.C. has received funding from the European Research Council (ERC) under the European Union’s Horizon 2020 research and innovation programme under grant agreement no. 756194 (ENERGYA). J.G. is supported by a research grant from Science Foundation Ireland (SFI) and the National Natural Science Foundation of China (NSFC) under the SFI-NSFC Partnership Programme, grant no. 17/NSFC/5181. D.P.v.V., R.S. and D.E.H.J.G. are supported by the Horizon 2020 NAVIGATE project, and D.P.v.V., R.S. and D.E.H.J.G. also acknowledge support from the COMMIT and Horizon 2020 ENGAGE project. F.P. acknowledges support through the project ENGAGE funded in the framework of the Leibniz Competition (SAW-2016-PIK-1), as well as through the project CHIPS, part of AXIS, an ERA-NET initiated by JPI Climate, and funded by FORMAS (SE), DLR/BMBF (DE, grant no. 01LS19XXY), AEI (ES) and ANR (FR) with cofunding by the European Union (grant no. 776608). R.S. acknowledges the financial support from the National Council for Scientific and Technological Development (CNPq), from the National Institute of Science and Technology for Climate Change Phase 2 under CNPq grant no. 465501/2014-1 and the National Coordination for High Level Education and Training (CAPES) grant no. 88887.136402/2017-00, all from Brazil. A.M. acknowledges support from the US Department of Energy, Office of Science’s Integrated Multisector Multiscale Modelling project and National Science Foundation’s Water Sustainability and Climate grant no. 1360445. This work was authored in part by the National Renewable Energy Laboratory (A.M.), operated by Alliance for Sustainable Energy, LLC, for the US Department of Energy (DOE) under Contract No. DE-AC36-08GO28308. S.F. is supported by the Environment Research and Technology Development Fund (2-1908 and 2-2002) provided by the Environmental Restoration and Conservation Agency, Japan. C.P. is supported by Korea Environment Industry & Technology Institute (KEITI) through Climate Change R&D Programme, funded by the Korea Ministry of Environment (MOE) (2018001310003).
Author information
Authors and Affiliations
- Copernicus Institute of Sustainable Development, Utrecht University, Utrecht, the Netherlands Seleshi G. Yalew, David E. H. J. Gernaat, Ioanna Mouratiadou & Detlef P. van Vuuren
- Water Systems and Global Change Group, Wageningen University, Wageningen, the Netherlands Seleshi G. Yalew, Michelle T. H. van Vliet & Fulco Ludwig
- Policy Analysis, Department of Multi-Actor Systems, Technical University of Delft, Delft, the Netherlands Seleshi G. Yalew
- Department of Physical Geography, Utrecht University, Utrecht, the Netherlands Michelle T. H. van Vliet
- Netherlands Environmental Assessment Agency-PBL, The Hague, the Netherlands David E. H. J. Gernaat & Detlef P. van Vuuren
- Advanced Science Research Center, GC/CUNY, New York City, NY, USA Ariel Miara
- National Renewable Energy Laboratory, Golden, CO, USA Ariel Miara
- Department of Landscape Architecture, College of Urban Science, University of Seoul, Seoul, Korea Chan Park
- International Institute for Applied Systems Analysis-IIASA, Laxenburg, Austria Edward Byers
- Fondazione CMCC, Venice, Italy Enrica De Cian & Shouro Dasgupta
- Università Ca’ Foscari Venezia, Venice, Italy Enrica De Cian & Shouro Dasgupta
- Potsdam Institute for Climate Impact Research, Leibniz Association, Potsdam, Germany Franziska Piontek & Robert Pietzcker
- Joint Global Change Research Institute, Pacific Northwest National Laboratory, College Park, MD, USA Gokul Iyer, Mohamad Hejazi & Silvia R. Santos da Silva
- MaREI Centre, Environmental Research Institute, University College Cork, Cork, Ireland James Glynn
- Institute for Sustainable Resources, University College London, London, UK Olivier Dessens
- Programa de Planejamento Energético, COPPE, Universidade Federal do Rio de Janeiro, Rio de Janeiro, Brazil Pedro Rochedo & Roberto Schaeffer
- Center for Social and Environmental Systems Research, National Institute for Environmental Studies, Tsukuba, Japan Shinichiro Fujimori
- Department of Environmental Engineering, Kyoto University, Kyoto, Japan Shinichiro Fujimori
- Laboratoire d’économie appliquée de Grenoble, Grenoble, France Silvana Mima
- Department of Atmospheric and Oceanic Science, University of Maryland, College Park, MD, USA Silvia R. Santos da Silva
- Council on Energy, Environment and Water, New Delhi, India Vaibhav Chaturvedi
- Laboratoire des Sciences du Climat et l’Environnement-LSCE, Paris, France Robert Vautard
- Seleshi G. Yalew